Latest from 5G/6G & Fixed Wireless Access/Mobile Evolution
What’s Next: Enhanced mobile broadband (eMBB), Ultra-reliable low-latency communications (URLLC) and Massive machine-type communications (mMTC) —
Known as the Advanced Mobile Phone System (AMPS) in the US, the first generation of mobile communications was analog cellular, used primarily for voice-only communications, but capable of up to 14.4 Kbps as a modem. Call quality was often poor at the margin of network coverage, often characterized by increasing static as one left a coverage area.
The second generation of mobile communications brought digital communications to cellular and much better voice call quality. Launched initially in Europe as the Group Special Mobile (GSM) standard, 2G would also be launched in the US as both GSM and the so-called "digital AMPS" standard as a digital extension of AMPS in the same frequency band.
One of the notable capabilities added with 2G was text messaging, often referred to as Short Messaging Service (SMS), which was available as a two-way (mobile origination and termination) day-one with GSM but would be initially terminate-only initially on D-AMPS systems. It is also important to note that 2G systems were also used to great effect in support of machine-to-machine (M2M) communications involving telemetry applications such as smart meter reading.
While there were some interim steps between 2G and 3G, such as the GSM focused General Packet Radio Service (GPRS) ranging from 20 to 40 Kbps, standardization towards the Universal Mobile Telecommunications Service (UMTS). The vision of third generation cellular would ultimately be in the order of 200 Kbps and realized by CDMA technologies such as Wideband Code Division Multiple Access (W-CDMA), planned as part of the evolution of GSM, and the CDMA2000 standard, which was part of the evolution of CDMA-based 2G networks.
The Long Term Evolution (LTE) standard for fourth generation cellular is currently the preferred technology for users globally as it provides substantially faster bandwidth than 3G, ranging from 150 Mbps to up to 1 Gbps with the LTE Advanced variation of the standard.
InvisiLight® Solution for Deploying Fiber
April 2, 2022Go to Market Faster. Speed up Network Deployment
April 2, 2022Episode 10: Fiber Optic Closure Specs Explained…
April 1, 2022Food for Thought from Our 2022 ICT Visionaries
April 1, 2022In addition to providing substantial data speed improvements, LTE also introduced the opportunity to support an end-to-end, all Internet Protocol based connection covering everything from the network core to the radio interface. To support this effort, and coincident with LTE deployment were some big changes to carrier core networks including introduction of IP Multimedia Subsystem (IMS). Among other things, IMS is very important for Voice over LTE (VoLTE).
With the introduction of LTE, the process of "sun-setting" 2G networks began in earnest as 3G is used for M2M (rather than 2G) and as a default back-up for locations in which LTE coverage is poor.
5G Sets the Stage
5G will be significantly faster than LTE, anticipated to achieve up to 100 Gbps with much lower latency due to faster overall throughput when deployed in conjunction with Mobile Edge Computing (MEC) equipment. It is difficult to talk about 5G without discussing MEC.
MEC is beneficial for LTE, but virtually essential for certain aspects of 5G networks. This is because mobile edge computing facilitates optimization of 5G network resources including focusing communications and computational capacity where it is needed the most. Without MEC, 5G would continue to rely upon backhaul to centralized cloud resource for storage and computing, diminishing much of the otherwise positive impact of latency reduction enabled by 5G.
In terms of the radio access network (RAN), there are some substantial challenges to overcome with 5G RAN. For example, the 5G new radio component that leverages millimeter wave (mmWave) RF has a high degree of attenuation (loss over the air and when hitting solid objects) than lower frequencies used in LTE. The higher frequency mmWave RF is largely line of sight based because of the high degree of fading. This works great for point-to-point applications, such as fixed wireless, but represents a significant challenge for mobility-related applications.
Physics dictates that higher frequencies need more power and/or more coverage as an RF signal fades more than a lower frequency signal. Since there are industry regulations that dictate maximum power, there will be a need for many more antennas for higher frequencies used with 5G as compared to LTE. In fact, there will need to be at least an order of magnitude more antennas than required for LTE.
In addition, the industry needs to deploy 5G smart antennas with beam-forming and Multiple Input / Multiple Output (MIMO) capabilities to deal with attenuation and to direct RF energy beamed from 5G antennas where and when it is needed the most. It is important to note that MIMO and other smart antenna technologies have existed prior to 5G but are being optimized to deal with the need to support mobile applications leveraging higher frequencies and substantially more cellular base stations. The use of beam-forming (beam steering) will be particularly important as a tool to focus high frequency RF signals into narrow beams to reduce interference and amplify the signal between base station and device
To support ample coverage and seamless mobility, 5G smart antennas will be found virtually everywhere in metropolitan areas, supporting a wide range of applications that are vitally important for certain applications such as self-driving cars and connected vehicle services.
While 5G deployment is leveraging some new frequency bands and will entail expansion of number of cells, much of it will NOT be mmWave. Instead, a big part of the 5G applications market is driven by the evolution of LTE in support of Internet of Things (IoT) networks and systems.
IoT solutions will benefit greatly from the implementation of 5G as cellular providers deploy Low Power WAN (LPWAN) IoT network capabilities. Initial deployments of IoT LPWANs have been non-cellular solutions based on proprietary technologies. In fact, the implementation of lower bandwidth in the sub-6 GHz range in support of IoT will lead to "sun-setting" of 3G networks for M2M communications. For example, AT&T has said it plans to discontinue 3G service in 2022.
5G applications, services, and solutions are typically referred to by the following categories:
• Enhanced mobile broadband (eMBB): Mobile broadband based apps including video watching, browsing, Mobile Office/Productivity, and cloud-based gaming.
• Ultra-reliable low-latency communications (URLLC): Augmented Reality, Virtual Reality, Telepresence (includes Holographic calling), Teleoperation/Tele-robotics, Autonomous Vehicles, UAV/Drone Operation, Public Safety, Smart Buildings and other latency-sensitive applications
• Massive machine-type communications (mMTC): 5G will facilitate a highly scalable M2M network for many IoT applications, particularly those that do not require high bandwidth.
One of the more important challenges with 5G is juggling multiple requirements found within each category, which are also often mutually exclusive. For example, URRLC applications require high reliability (e.g., works exactly as it is supposed to work exactly when it’s needed) yet also require very low latency and very high bandwidth. Achieving all 3 of these in support of quality of service and quality of experience requirements for demanding customers such as smart factories will be a major challenge for service providers.
In terms of network deployment and operations, public versus private networks is an increasingly important consideration with 5G. More specifically, business customers that previously relied upon carrier-based, publicly available service offerings will instead choose private networks.
While some business customers will vie for virtualized instances of carrier infrastructure/platforms, other enterprise, industrial, and government customers will go with private networks within their own control and/or facilities. In other words, some of these private networks are going to be in competition with carrier communication services as the likes of Nokia are actually competing with their own customers (e.g., carriers are customers of infrastructure providers like Nokia, Ericsson, etc.).
Like this Article?
Subscribe to ISE magazine and start receiving your FREE monthly copy today!
While many of these changes in public versus private networks currently impact the radio access network and edge computing alone (e.g., businesses still need connectivity with carriers for WAN communications), it sets the stage for potential evolution towards a more distributed service realization environment that may involve a more dispersed core network that is not completely owned/controlled by the legacy carriers.
For more information about 5G, see What Will and Won’t Happen with Fifth Generation Cellular
Be sure to check out Mind Commerce’s new offerings to ISE’s current customers for syndicated and custom research to help solidify your company’s product roadmap. See https://isemag.com/research/ for more information.
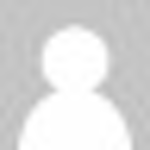